3b. Pitot tube drain blocked first; then ram air blocked; static port remains unobstructed.
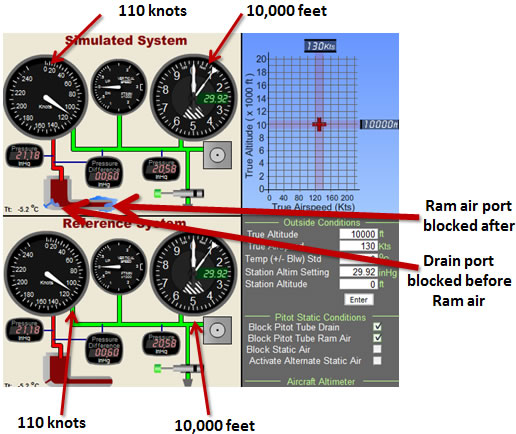
Fig 4-32 Pitot tube drain blocked followed by ram air port at 10,000 feet and at a true airspeed of 130 knots.
Suppose an aircraft is flying at 10,000 feet and is at a true airspeed of 130 knots. The Pitot tube drain then becomes blocked, followed by the ram air port (Fig 4-32). Since the static port remains unobstructed, there is no effect in either the VSI or altimeter. If the aircraft maintains altitude the pilot will not notice any changes in the airspeed indicator even if airspeed changes the airspeed. In this situation, because the air in the Pitot tube chamber is trapped, the pressure (21.18 inHg) will remain the same in this chamber regardless of any airspeed for altitude change. Since the airspeed indicator measures the difference in pressure between the air in this chamber and the static air line, the airspeed indicator will now move when altitude changes because the static pressure will change with altitude.
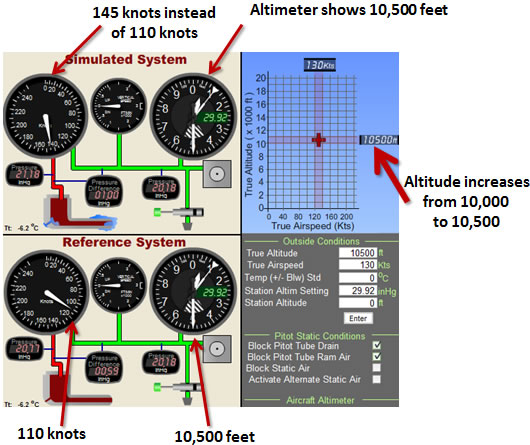
Fig 4-33 Aircraft climbs 500 feet and maintains airspeed.
Let's see what happens when the aircraft climbs 500 feet to 10,500 feet (Fig 4-33). Because the static air pressure decreases with altitude the airspeed indicator will be measuring a greater difference in pressure (21.18 inHg of the chamber and 20.18 inHg of the static air line). The resulting difference of 1.00 inHg corresponds now to approximately 145 knots instead of what it should be showing (110 knots). Looking at the reference system we can confirm that at the correct difference: qc = PT - P = 20.77 - 20.18 = 0.59 inHg, the indicated airspeed should be 110 knots (see chart 2-3). In other words, in just 500 feet, the difference in airspeed is 35 knots (assuming that true airspeed of the aircraft has not changed, which it hasn't in this example). Notice how the direction in which the airspeed changes is in the opposite direction of scenarios 1a, 1b, 1c and 3a. This is why this situation may be particularly dangerous. Pilots are used to having their airspeeds decrease when pulling back on the yoke which usually results in an increase in altitude. Here the pilot may pull back on the yoke and since the airspeed indicator would show an increase in airspeed, the pilot may pull the yoke back even further. This may lead the aircraft into a stall or loss of control before the pilot recognizes there's a problem, quickly!
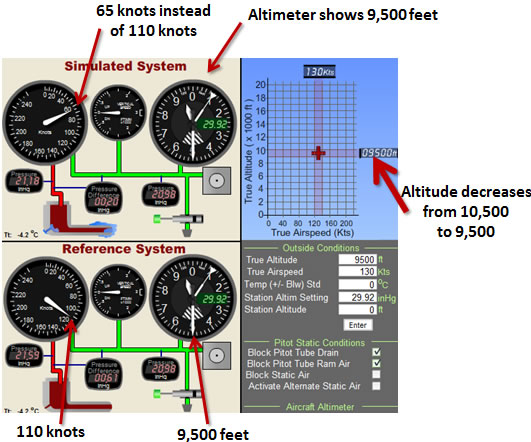
Fig 4-34 Aircraft descends 1000 feet and maintains airspeed.
Here we have the aircraft descending from 10,500 to 9,500 feet (Fig 4-34). As expected, the indicated airspeed decreases. It is said that in this type of blockage situation the airspeed indicator will have an altimeter like behavior. In other words the indication will rise if the altitude increases and will lower if the altitude decreases. Notice that the difference between the air pressure in the Pitot tube chamber (21.18 inHg) and the static air line (20.98inHg) which is now greater, is 0.20 inHg. 65 knots (see chart 2-3) is the indicated airspeed that corresponds to that difference in pressure. Here the altitude of 500 feet below the altitude at which the blockage occurred produces a difference of 45 knots (once again, assuming that true airspeed of the aircraft has not changed, which it hasn't in this example). One of the dangers here is that the pilot sees a decrease in airspeed, pushes the yoke even more forward causing the aircraft to descend more which makes the indication of the airspeed indicator decrease further. The aircraft can easily overspeed, lose control or crash in the terrain below if the pilot does not recognise the problem.
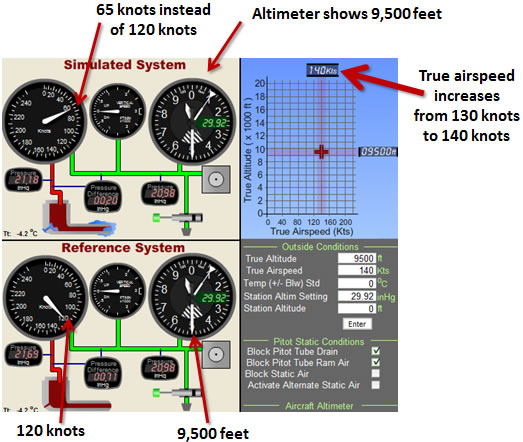
Fig 4-35 Aircraft maintains altitude and increases airspeed by 10 knots.
If the aircraft maintains altitude and increases its true airspeed to 140 knots there is absolutely no effect in the airspeed indicator (Fig 4-35) since airspeed has no effect when the ram air is blocked.
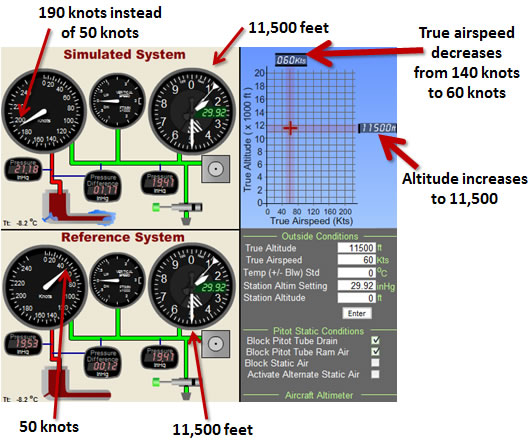
Fig 4-36 Aircraft climbs 2000 feet and reduces true airspeed by 80 knots.
Here in figure 3-36 we have what may happen if the pilot were to pull back on the yoke (causing a climb) in attempt to slow down the aircraft. The true airspeed could slow down to 60 knots as the aircraft climbs to 11,500 feet while the pilot would see an indication of 190 knots instead of the correct indication (see reference system) of 50 knots. The 190 knots (see chart 2-3) corresponds to the difference in pressure of 1.77 inHg between the Pitot tube chamber (21.18 inHg) and the static air line (19.41 inHg).
If the static port also gets blocked this scenario becomes scenario 1d and the airspeed indicator, VSI and altimeter will freeze at their current indications.
|